Planet and Satellite Formation
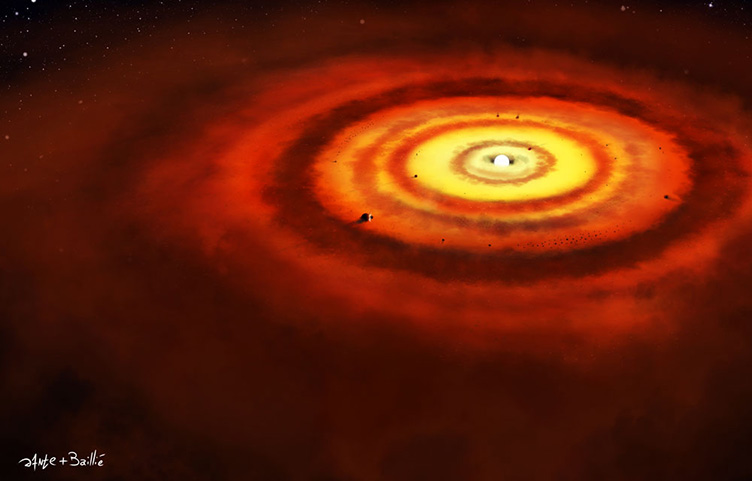
Modeling the hydrodynamical evolution of protoplanetary disks
Protoplanetary disk observations reveal a diversity of thermodynamical and geometrical structures which origins we have to understand in order to constrain the formation scenarios of the solar system. We follow the long-term evolution of such disks, from their birth in the gravitational collapse of the primordial molecular cloud to their evaporation due to the intense stellar irradiation. It is therefore necessary to consider jointly the numerous physical effects that drive this evolution with different timescales: the collapse of the molecular cloud, the stellar evolution, the disk thermodynamical evolution, its viscous spreading, the photo-evaporation...
Based on numerical hydrodynamical simulations, we model consistently the disk evolution, its temperature structure (involving a competition between viscous heating and irradiation heating) and the shape of its surface. In addition, the thermal model accounts for the composition of the disk dust through an opacity model that reflects the dust sublimation as a function of the disk midplane temperature. Our approach rests on the coupling between the disk thermodynamics, its geometrical structure, its chemical composition and its dynamical viscosity. This allowed to retrieve the main observational characteristics of evolved protoplanetary disks. Moreover, we showed that disks can no longer be simply modeled with power-law profiles as they host shadowed regions (that are not irradiated), related to the temperature plateaux located at the dust sublimation lines.
Trapping planetary embryos
Though the timescale of planet formation is about the same order of magnitude as disk typical lifetime (a few million years), protoplanets should spiral inward by type I migration and fall onto their host star in a few 100,000 years. Based on our disk evolution model, we showed that density and temperature bumps directly reflect in variations and possible canceling or reversing of the migration: in particular, we showed that the dust sublimation lines could generate such “planet traps” that save protoplanets and favor their accumulation on specific orbits and therefore their growth by collisions and accretion. The locations of those traps depend not only on the disk evolution but also on the planet growth: planets that grow too fast are more likely to escape the traps and fall on the central star by resuming inward migration. Alternatively, they may open a gap in the disk and begin type II migration.
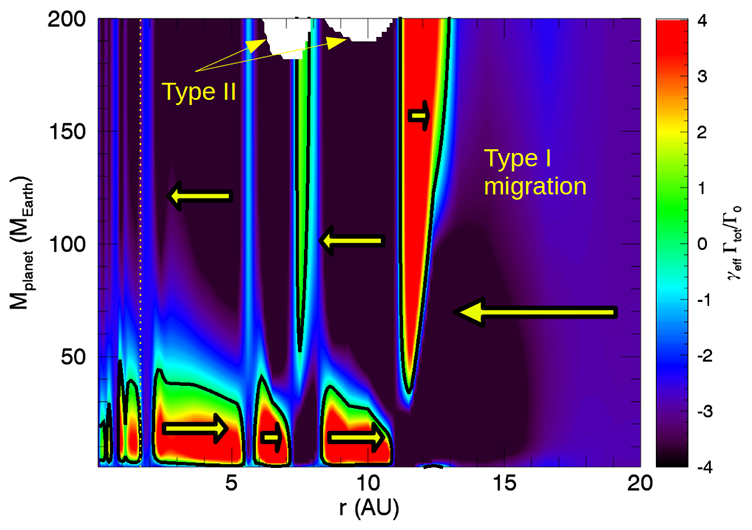
Forming the first solids: determination of the favorable conditions
Calcium-Aluminum rich Inclusions (CAIs) that can be found in some of the most primitive meteorites are the oldest solids that we know of in the solar system. They may have formed in a few thousand years at the very beginning of the formation of the solar system, in the innermost regions of the protoplanetary disk, where temperature and pressure conditions would allow the condensation, partial melting and coagulation of dust grains. As these regions cannot be observed directly in distant protoplanetary disks, CAIs remain the best tracers of the conditions that used to rule the inner protosolar disk.
The relationship between astrophysics and cosmochemistry naturally appears here since the thermodynamical and dynamical conditions of the formation of the first solids are directly inherited from the disk itsself. Thus, coupling a hydrodynamical code for the disk evolution with a Lagrangian code for the dust grain transport, we followed the thermal and dynamical history of growing CAIs. In particular, we showed that the number of successive heating episodes may determine the main characteristics of the formed CAIs, and therefore their family.
Minimum Mass Solar Nebula (MMSN)
Based on numerical simulations coupling the young Sun evolution with the formation of the surrounding protoplanetary disk, we find that the MMSN is equivalent to a 3 Myr-old disk.
References
- Morbidelli, A., Baillié, K., Batygin, K., Charnoz, S., Guillot, T., Rubie, D., Kleine, T., 2022. Contemporary formation of early Solar System planetesimals at two distinct radial locations. Nature Astronomy 6, 72.
- Baillié, K., Marques, J., Piau, L., 2019. Building protoplanetary disks from the molecular cloud : redefining the disk timeline. Astronomy & Astrophysics 624, A93
- Baillié, K., Charnoz, S., Pantin, E., 2016. Trapping planets in an evolving protoplanetary disk: preferred time, locations and planet mass. Astronomy & Astrophysics 590, A60
- Baillié, K., Charnoz, S., Pantin, E., 2015. Time Evolution of Snow Regions and Planet Traps in an Evolving Protoplanetary Disk. Astronomy and Astrophysics 577 [Press Release]
- Charnoz, S., Aléon, J., Chaumard, N., Baillié, K., Taillifet, E., 2015. Growth of Calcium-Aluminum-rich inclusions by coagulation and fragmentation in a turbulent protoplanetary disk. Icarus 252
- Baillié, K. & Charnoz, S., 2014. Time Evolution of a Viscous Protoplanetary Disk with a Free Geometry: Toward a More Self-consistent Picture. Astrophysical Journal, 786
Last update Wednesday 18 December 2024