Dynamical planetology
For about ten years, the increase in the accuracy of astrometric measurements, as well as the enlargement of the time span on which the observations are available, have necessitated to refine the dynamic modeling of the systems studied. This is particularly true for natural satellites and asteroids. Thus, the dissipation of energy in the form of heat due to the tides in the planets and their satellites, or the thermal inertia of the asteroids are all effects that must be taken into account today to understand the movement of these objects. Where astrometry gave mostly only masses and sometimes some additional gravitational coefficients of these objects, it is now possible to provide additional data of primary importance to better understand the interior of these objects, their evolution and even their formation.
Tidal effects
The effects of tides are due to the differential gravitaional attraction of one body to the different portions of another. The body undergoing these tides will then take an ellipsoidal shape, a bit like that of a rugby ball. In doing so, the deformed object sees its gravitational field modified, thus printing a different movement to those around it.
Our team is very involved in the characterization of these effects through its strong involvement in the ENCELADE international team. In 2009 (Lainey et al., Nature), we showed that the satellite Io could be close to thermal equilibrium, the heat evacuated at its surface being compensated at the same time by the one generated by internal friction under the action of tidal deformations. This result implies the existence of a sufficiently efficient heat transport mechanism inside the satellite, while imposing a viscosity strong enough to produce the large amount of heat observed at the surface.
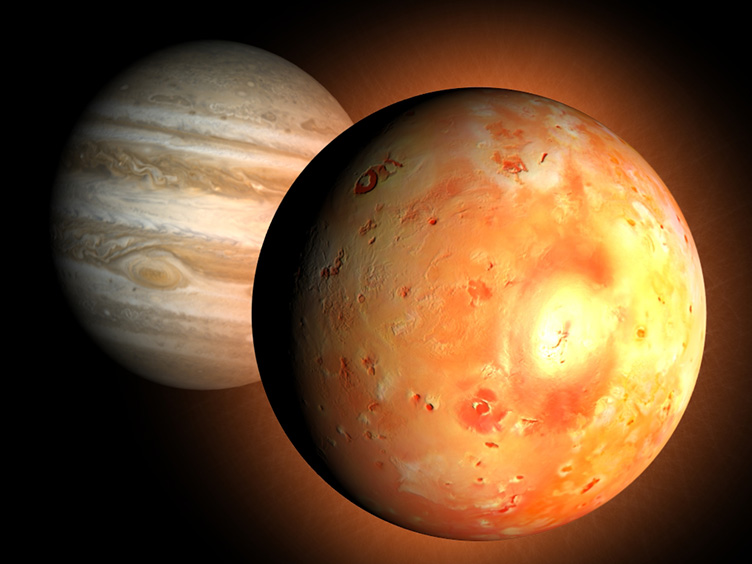
More recently, our team has been involved in quantifying tidal effects within Saturn. If the tides are now visible on Encelade, via the presence of geyser on its south pole, the tides within the planet itself seem to be a major player in the physics of this system. Indeed, the dissipation of energy inside planet Saturn induces an orbital expansion of the moons over time. The long-term evolution of this system is therefore directly correlated to the friction present in the core of the planet.
We have shown in 2012 (Lainey et al., ApJ) and in 2016 (Lainey and al., Icarus) that the amount of energy released in the form of heat inside planet Saturn, that is associated with tides raised by each of the moons, was ten to one hundred times higher than beleived before. This result implies that the moons we observe today could be much younger than the age of formation of the solar system (4.5 billion years).
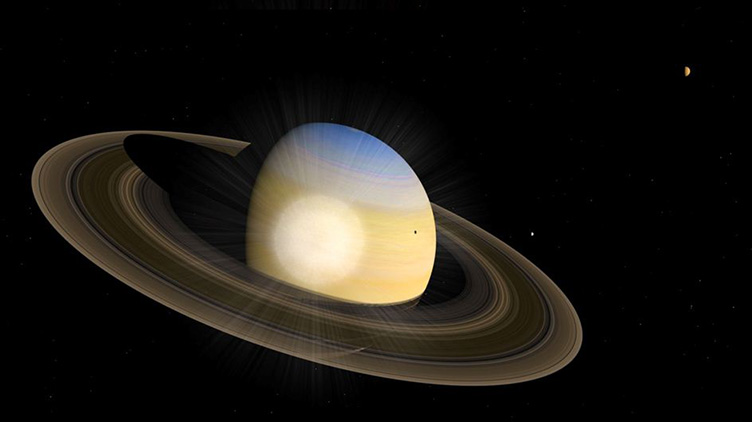
In 2016 we also managed, for the first time, to quantify by observations the deformation of the gravitational field of a giant planet under the action of the tides it undergoes. This result was made possible by the presence of coorbital moons to the main satellites Tethys and Dione. Indeed, the latter have the distinction of being permanently framed at 60° along their orbit by two other smaller satellites. By this "off-axis" position, the movements of the small moons are modified by the deformation of Saturn, generated itself by the tidal forces exerted by Tethys and Dione. As tiny as they are, these movements are measurable because, the system remaining at constant geometry, the effects accumulate over time, until producing variations of a few tens of kilometers over ten years.
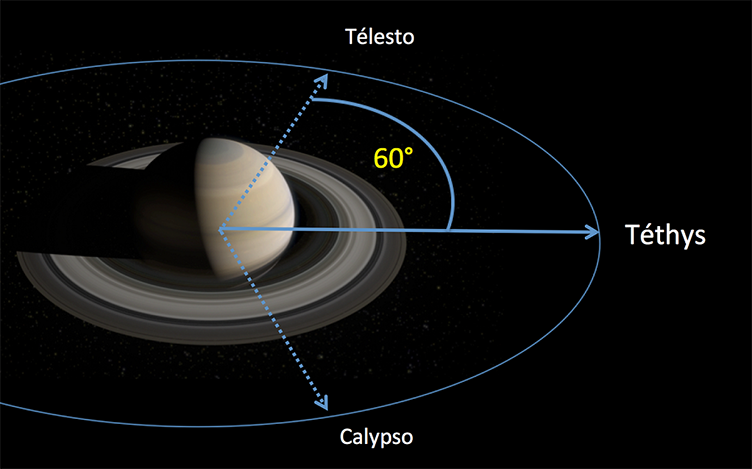
Asteroids and Yarkovsky effect
Asteroids and small bodies in general are no longer simple point masses in motion, as one might consider in classical celestial mechanics approach. Their dynamic study now requires to take into account planetary aspects. Conversely the study of their orbits and dynamics brings information on their (macroscopic) physical properties. Our team has developed models for the analysis of ground- and space-based data, taking into account astrometric data supplemented by other measurements. We were able to participate in various research groups and consortiums dealing with the dynamics of small bodies from the near-Earth to trans-Neptunians regions.
Asteroids masses, bulk-densities, and internal structures
The space mission Gaia brings and will bring considerable advances in our knowledge of small solar system bodies. Gaia observes natural satellites, comets, asteroids and asteroids’ satellite. We are also involved in accompanying ground-based observations, including the Gaia-FUN-SSO network. Gaia, thanks to its high observations’ quality and astrometric accuracy, makes it possible to improve the determination or the adjustment of orbits in a spectacular way (with gain of a factor of about 50).
Asteroid-asteroid close encounters, as well as the analysis of binary satellite (or multiple systems) orbits, make it possible to determine the masses of these bodies, their density, and their porosity (Kovalenko et al., 2016, IAUs, Hestroffer et al. 2014, DDA). This information is otherwise accessible only during a rendez-vous by a space probe, or with a dedicated space mission, so for only very few targets. The knowledge of these physical properties is fundamental to better understand the formation processes, and the collisional or dynamical evolution of these primitive bodies, and of planetary systems in general. A large number of relatively small asteroids are believed to be gravitational aggregates with large macroscopic porosity. It appears that such objects are better modeled as granular systems; hence an international and interdisciplinary team led by us, gathering planetary sciences and granular media experts, has obtained the support of the ISSI institute (ISSI team NuMAGS - Asteroids as gravitationnal aggregates)
Additionally, Gaia allows local tests of general relativity or gravitation by measuring the deviations of measured accelerations from predictions (Hees et al., 2015, SF2A).
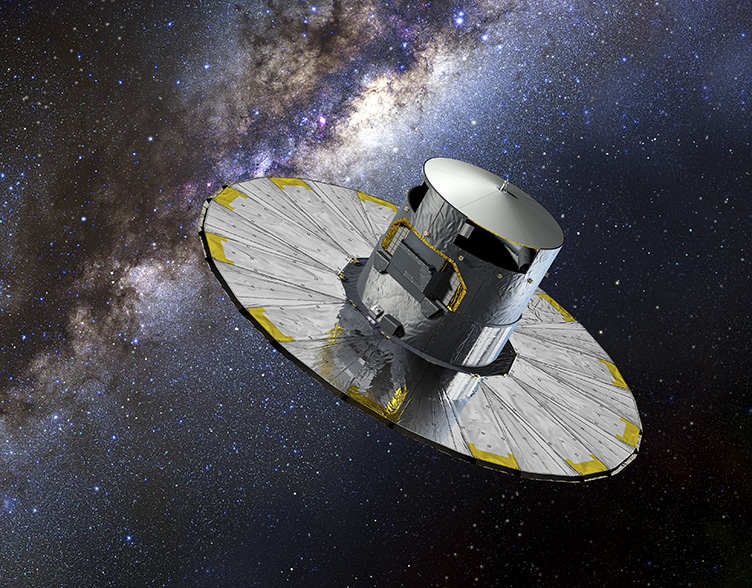
Near-Earth Objects and Yarkovsky effect
Long-term orbital propagation and near-Earth asteroid impact probability calculations are limited by our precise knowledge of the dynamic model, and in particular by modeling non-gravitational forces such as the Yarkovsky effect. This radiation force acts on small asteroids (from a few meters to a few hundred meters in size) and induces a weak acceleration to the object. In the short term, the Yarkovsky effect degrades our knowledge of the asteroid’s orbit, especially in the case of close encounters and probability of impact. In the long run, this effect partly structures the dynamic populations of asteroids.
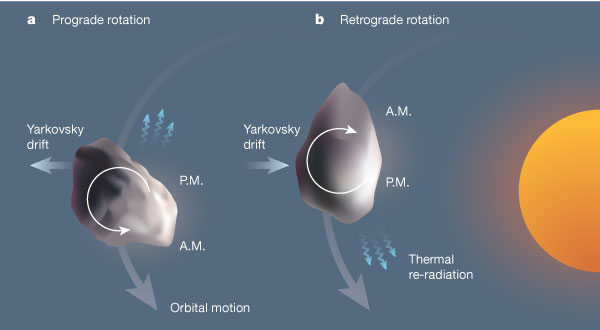
The modeling of the Yarkovsky effect involves a large number of physical parameters (such as density, obliquity, size, or thermal characteristics of the object) that are generally unknown or in the best case not loosely constrained. The induced acceleration produces mainly a small drift of the semi-major axis (of the order of a few hundred meters per year), which drift depends on these physical parameters. For some objects, it is possible to directly measure it from observational data. Thus, we have been able to measure a reliable semi-major axis drift for about fifty asteroids with a long observation period (over 20 years) or very precise radar measurements (Desmars A&A, 2015). The measurement of these drifts makes it possible to constrain the physical parameters related to such Yarkovsky effect, and to validate scenarios of asteroid population dynamics. For example, the sign of the drift in semi-major axis gives the rotation direction (prograde if the drift is positive, retrograde otherwise). Also, the transfer mechanism of asteroids from the main belt to orbits closee to the Earth (NEO) implies that 2/3 of the near-Earth asteroids have a retrograde rotation (therefore a negative drift); a ratio that is confirmed in the drifts that we could measure.
In the future, the Gaia mission will provide accurate astrometric measurements of asteroids as well as a stellar catalog, that will allow astrometric reduction of unpreceedented quality, making it possible to measure orbits drift for a large number of objects. This will improve our understanding of the Yarkovsky effect on NEOs dynamic and close encounters, constrain the physical parameters related to this effect, and validate asteroid population dynamic scenarios.
References
- Bancelin D., Colas F., Thuillot W., Hestroffer D., Assafin M. 2012. A&A 544, 15. Asteroid (99942) Apophis: new predictions of Earth encounters for this potentially hazardous asteroid
- Desmars J., 2015. A&A. 575, 53. Detection of Yarkovsky acceleration in the context of precovery observations and the future Gaia catalogue
- Desmars J., Bancelin D., Hestroffer D., Thuillot W. 2013. A&A, 554, 32. Statistical and numerical study of asteroid orbital uncertainty
- Gaia collaboraiton et al. 2016. A&A. 595, 1. The Gaia mission
- Hees A., Hestroffer D., Le Poncin-Lafitte C., David P. 2015. SF2A-2015, pp.125. Tests of gravitation with GAIA observations of Solar System Objects
- Hestroffer et al. 2017. Powder & Grains conf. in EPJ Web of Conf. Vol. 140, p. 14011. Small solar system bodies as granular systems
- Hestroffer D., David P., Hees A., Kovalenko I., Kudryashova M., Thuillot W., et al. 2015. DDA 46, #30.504. Aspects of Solar System Objects Dynamics with the Gaia Mission and in the Gaia Era
- Hestroffer D., Dell’Oro A., Cellino A., Tanga P. 2010. LNP 790, 250. The Gaia Mission and the Asteroids
- Kovalenko I, Stoica R., Emelyanov N. V., Doressoundiram A., Hestroffer D. 2016. IAUs 318, 244. Bayesian statistical approach to binary asteroid orbit determination
- Lainey V., Jacobson R. A., Tajeddine R., Cooper N. J., Murray C., Robert V., Tobie G., Guillot T., Mathis S., Remus F., and 8 coauthors 2017. Icarus, 281, 286. New constraints on Saturn’s interior from Cassini astrometric data
- Lainey V., et al. 2012. ApJ 752, 14. Strong Tidal Dissipation in Saturn and Constraints on Enceladus’ Thermal State from Astrometry
- Lainey V., Arlot J.-E., Karatekin Ö., van Hoolst T. 2009. Nature 459, 957. Strong tidal dissipation in Io and Jupiter from astrometric observations
- Müller M. et al. 2011. Icarus 205, 460 Eclipsing binary Trojan asteroid Patroclus: Thermal inertia from Spitzer observations
- Mouret S., Hestroffer D., Mignard F. 2008. P&SS 56, 1819. Asteroid mass determination with the Gaia mission: A simulation of the expected precisions
- Tanga et al. 2009. ApJ 706, 197. Rubble-Pile Reshaping Reproduces Overall Asteroid Shapes
Last update Friday 19 June 2020